Research Overview
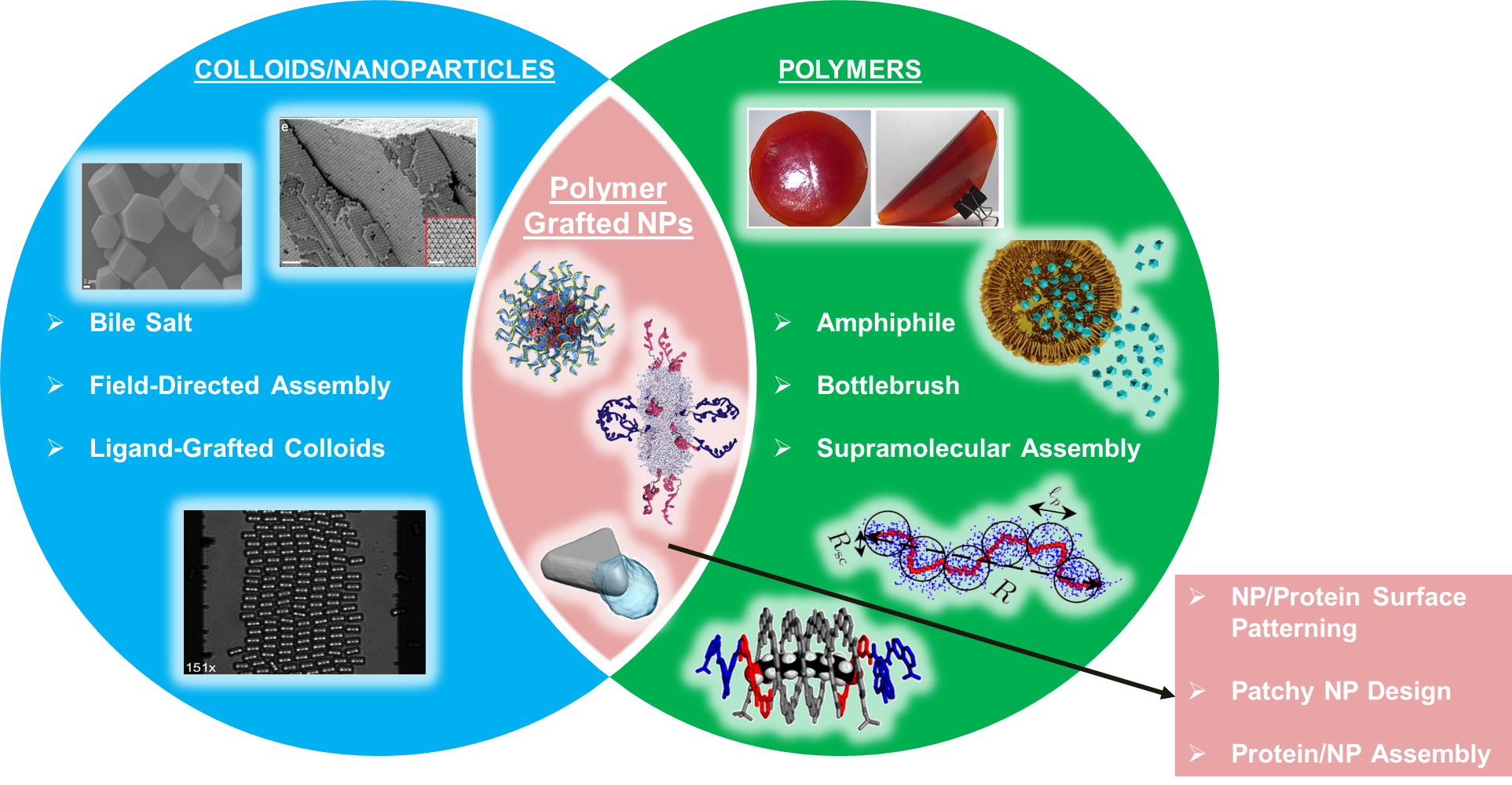
Over the last decade, there has been an explosive growth in our capability to synthesize nanoscale building blocks (NBBs). These NBBs possess a wide range of optical, structural, magnetic, and/or catalytic properties, suggesting that they can serve as the ideal design space for materials discovery spanning a diverse range of technologically open challenges.
Our group employs computational modeling and theory to develop strategies for designing colloidal, nanoparticle, and polymeric self-assembly involving combinations of NBBs into structures that exhibit multi-functional properties. Specifically, we aim to answer three broad questions:
1). How can we rapidly and accurately model novel complex building block monomers?
2). How can we understand and predict the self-assembly behaviors of NBBs?
3). How can we engineer experimentally realizable nanoscale and polymeric building blocks to target novel materials properties?
Research Projects
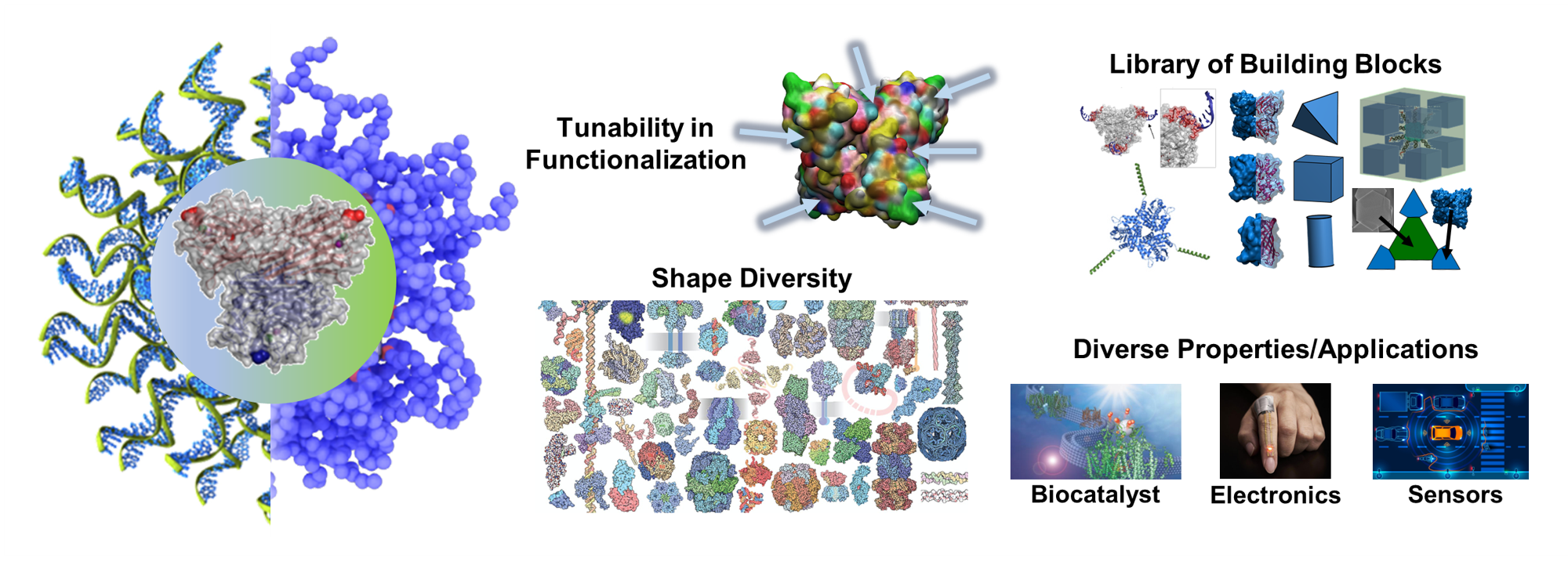
Polymer and DNA Functionalized Protein Self-Assembly
Polymer/DNA grafted proteins are a rapidly growing subclass of nanoscale building blocks. These synthetic-biological hybrids merge the natural diversity in protein functionalities with strategies that leverage polymer/DNA interactions to direct hierarchical self-assembly. As such, they serve as ideal building blocks for the creation of multifunctional biological composites, where proteins imbue the material with unique combinations of properties previously unattainable in synthetic systems and polymer/DNA grafts mediate network organization to enable materials fabrication at technologically relevant micron and millimeter length scales. Our group studies the fundamental physics underlying their assembly process and seeks to create a library of computationally validated building blocks for experimental synthesis.
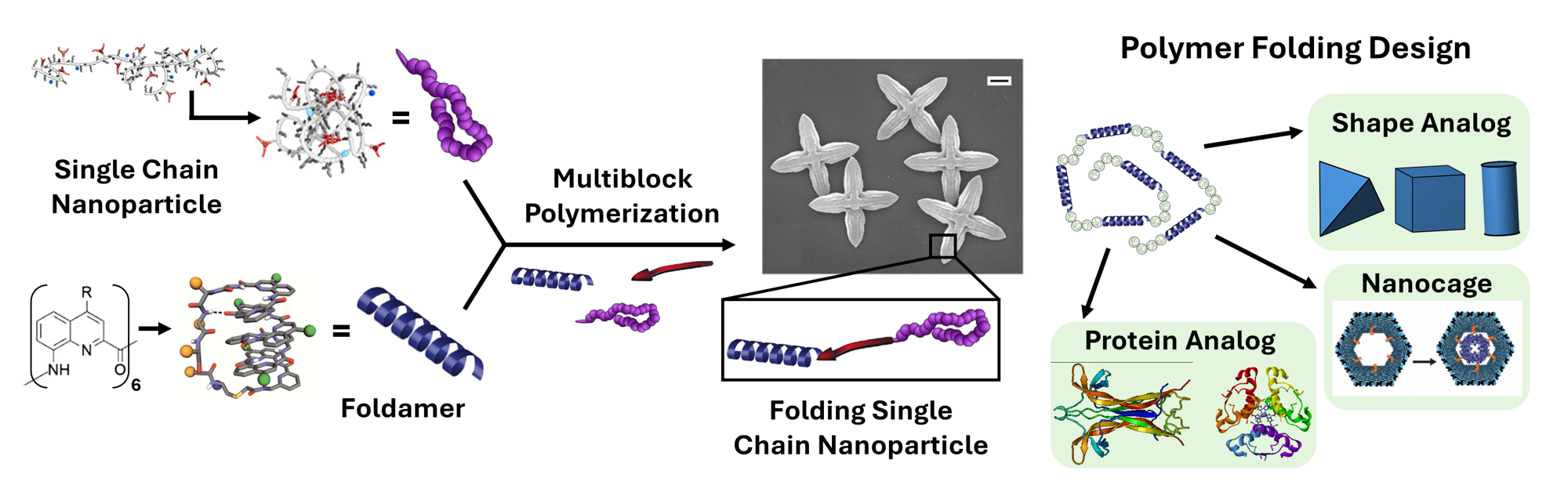
Polymer Origami
Current barriers in designing polymer self-assembly lie in the need to imbue knowledge of tunable interactions, self-limited orderings, and pathway engineering into a single folding process. While control over each individual component is possible, challenges associated with chain dispersity and kinetic traps are compounded when attempting to merge all components together. These barriers destabilize programmed interactions, ultimately disrupting chain folding. Our group aims to address this challenge by designing “folding polymers” – multiblock copolymers constructed from block polymerization of amorphous and foldameric units. These copolymers utilize the amorphous blocks to enhance backbone flexibility and foldameric blocks to define intrachain directional interactions needed for proper folding. This approach will be leveraged to create nanoscale catalysts, membranes, and reconfigurable materials.
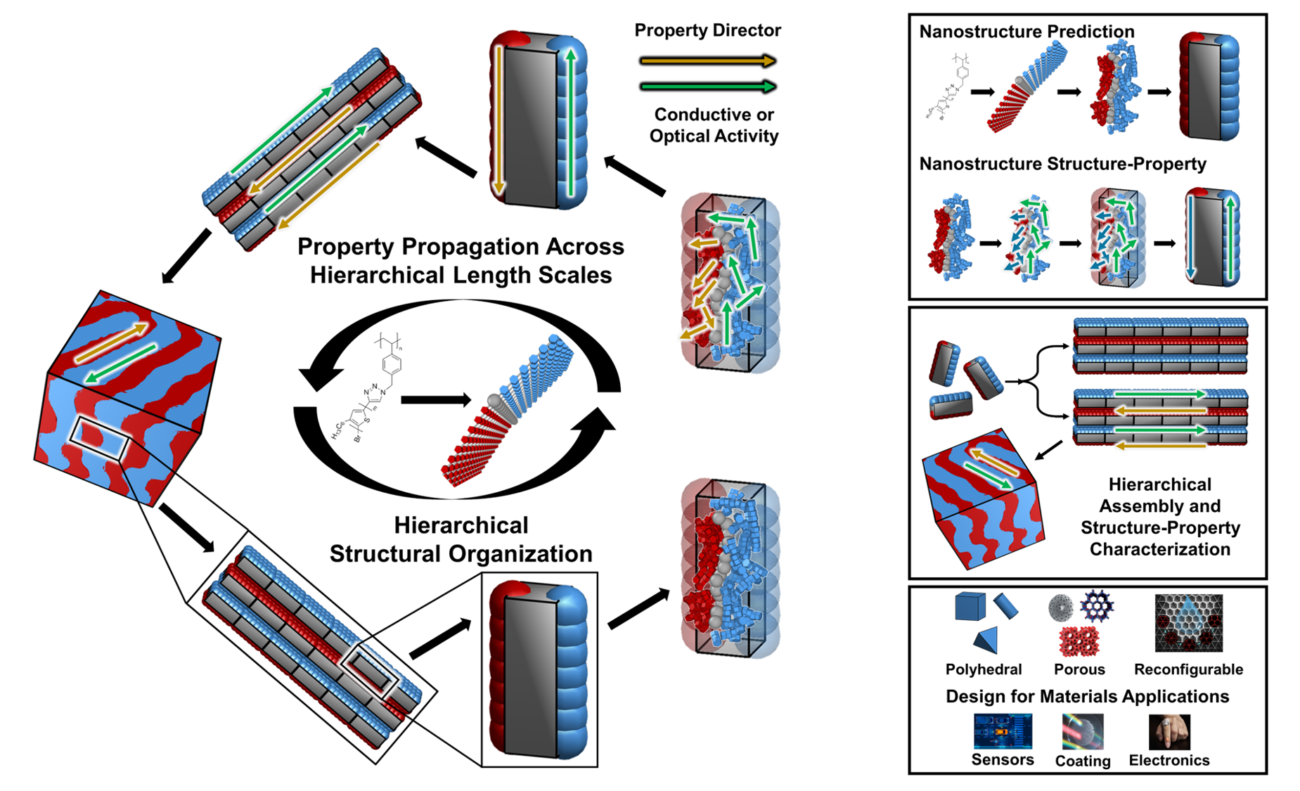
Structure-Property Relationships in Bottlebrush Polymers
Recent advances have enabled precision synthetic control of bottlebrush polymers. However, bottom-up designs of bottlebrush systems are currently limited due to a lack of fundamental understanding of how microscopic features specific to a single bottlebrush propagate to the mesoscale where emergent properties are a result of interactions between multiple polymers. Our group aims to map the structure-morphology-property relationships of bottlebrush polymers across all relevant self-assembly length scales. We will elucidate the underlying mechanisms governing the experimentally observed morphological and functional complexities intrinsic to this class of polymers. The fundamental insights obtained will be leveraged to define design principles for engineering hierarchical organization of bottlebrush polymers, where monomer-level spatial/orientational ordering within a single bottlebrush chain is directly coupled to inter-chain interactions and mesoscale-level morphologies.
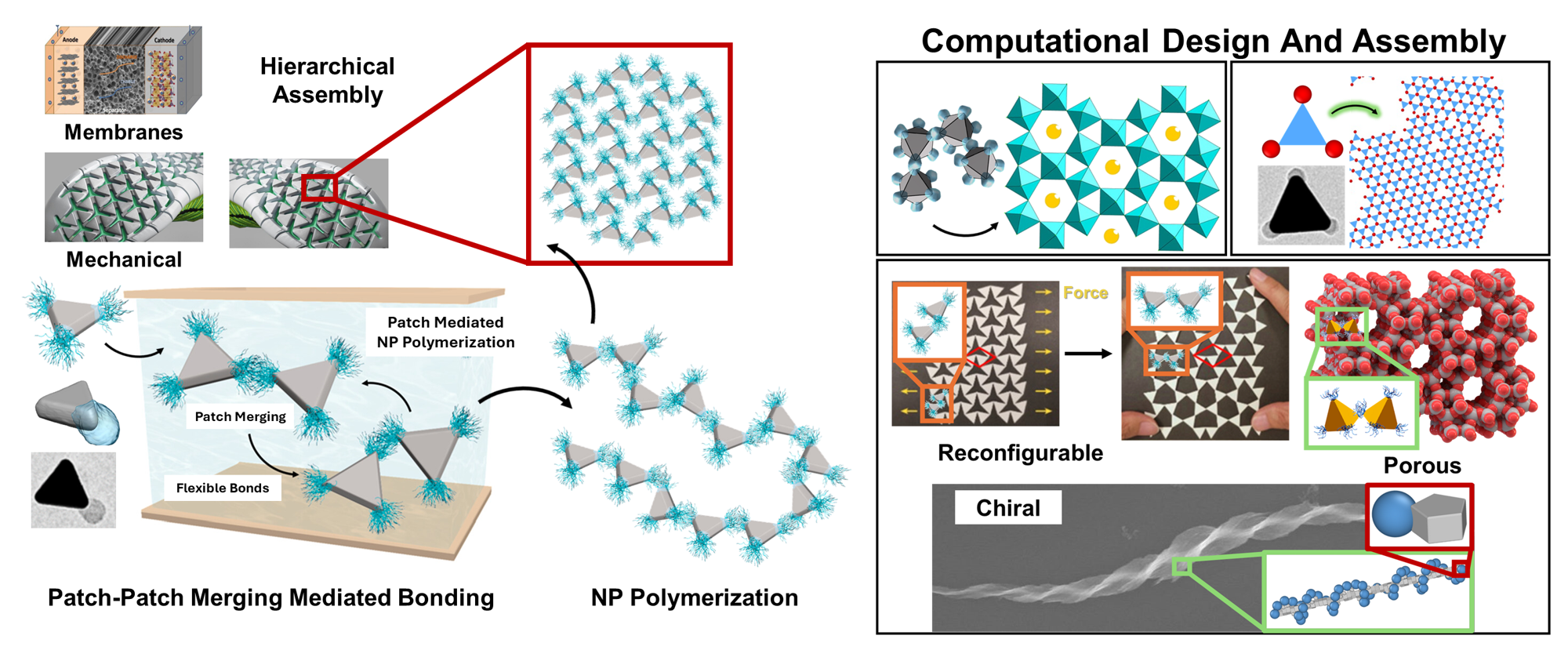
Computational Design of Macromolecular Polymers
Macromolecular polymers are created via polymerization of patchy nanoparticle subunits. Specifically relevant are nanoparticles with patchy polymer grafting that interact via surfactant-polymer complexation. These “‘polymers” blend the toughness and optical properties of inorganic nanoparticles with the flexibility and reconfigurability of polymeric patches, providing a route for multifunctional material design. Challenges in designings these polymers revolve around complexities arising from the interplay between flexible “bonds” that form due to patch merging and entropic interactions between nanoparticles. Our group seeks to understand the fundamental forces governing these interactions to design a computationally validated suite of macromolecular polymer candidates (as well as their patchy nanoparticle subunits) for use in making novel membranes, sensing, and reconfigurable materials.
Research Tools and Approaches
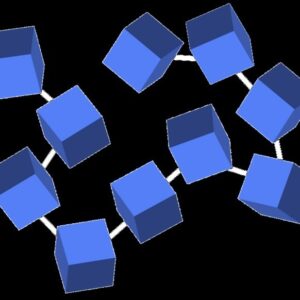
Computational Methods Development
We develop new computational algorithms for simulating complex building block geometries in Monte Carlo and Molecular Dynamics simulations.
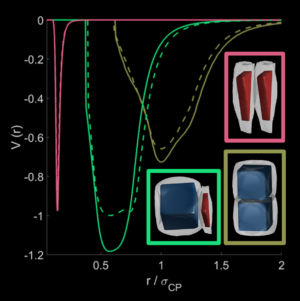
Coarse-Grained Force Field Parameterization
We focus on theory-driven and physics-based approaches to develop simple potentials/models for complex building blocks, enabling rapid simulations for a diverse range of colloids, nanoparticles, and polymeric systems.
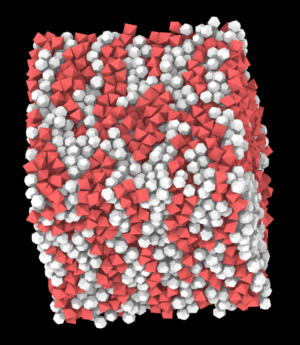
Computational Self-Assembly and Phase Behaviors
We employ our developed potentials to perform simulations of colloidal, nanoparticle, and polymer self-assembly and characterize their assembly pathways, phase behaviors, and materials properties.
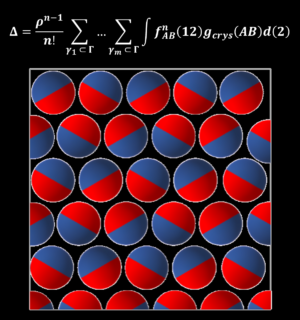
Theory Development for Assembly Prediction
We derive analytical theories to predict assembly morphologies and materials properties. Such theories are ideal for use in high-throughput computational screening of large design parameters space to guide experimental synthesis and explorations.